AZoRobotics speaks with Thomas P. Russell from the University of Massachusetts Amherst about the first self-driven, aqueous robot that works nonstop without electricity, inspired by the natural behavior of water-walking insects.
What benefits do robotic systems provide in laboratories?
Anytime you can get a system that can repeatedly and autonomously perform a function, you can alleviate a researcher from performing a monotonous task. Alternatively, you can get a system to perform a dangerous task without the need for a researcher, for example, a chemical reaction that produces toxic or explosive materials. I can also think of cases where you could use such repeated functions to generate or store energy.
What natural behavior inspired you to create an autonomous, aqueous-based synthetic system?
The natural behavior of water-walking insects, like the water striders and some insect larvae, inspired our research into creating an autonomous, aqueous-based synthetic system.
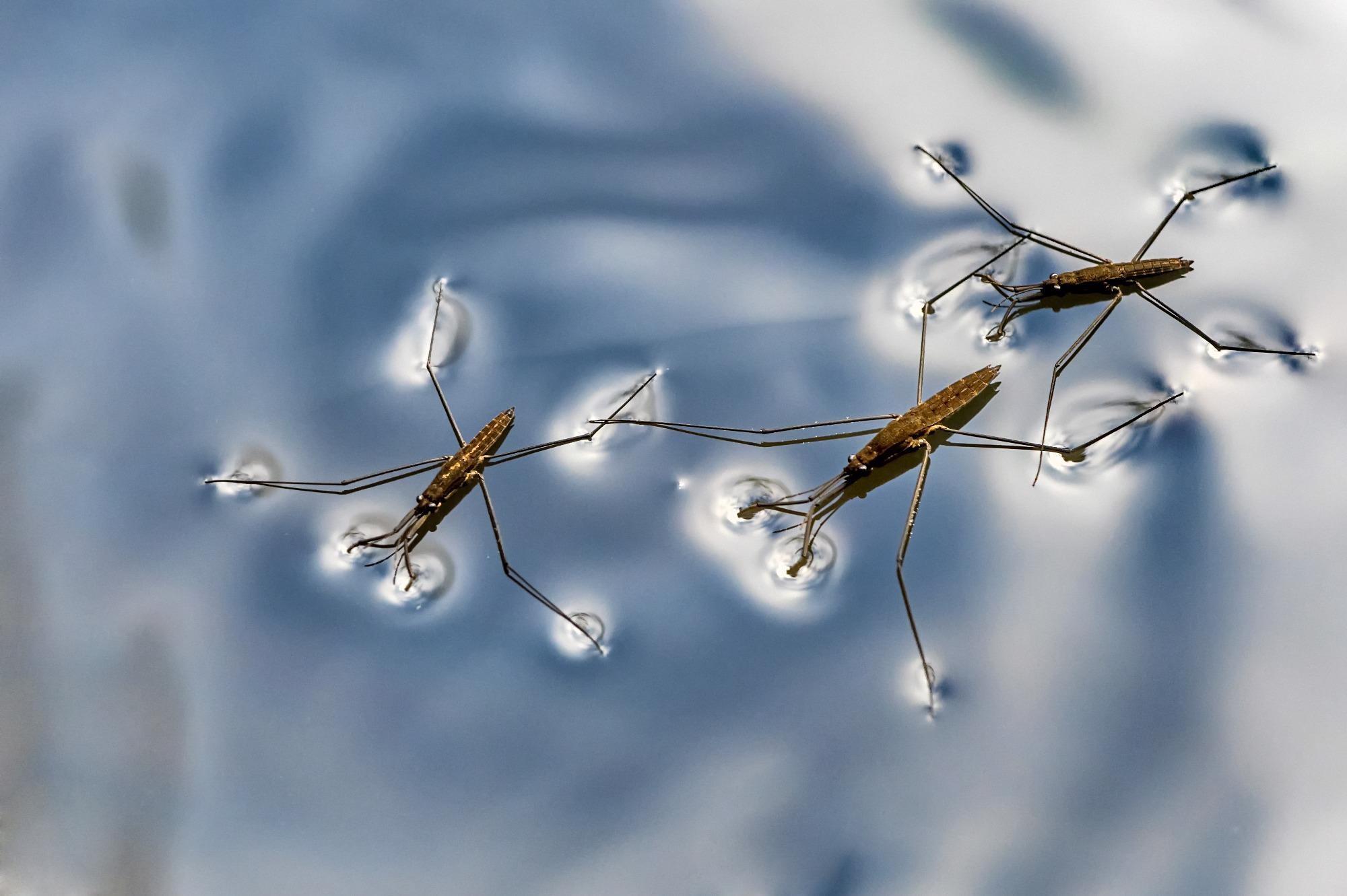
Image Credit: Shuttertsock.com/ Jan Miko
Why have robots that can work autonomously and continuously without electrical input never been established before?
Efforts have been focused, up to now, on mechanically driven systems. We have known about the influence of capillary forces on the ability to move objects on liquid surfaces for some time; in fact, it is termed the “Cheerio Effect”. Have you ever noticed how Cheerios in a bowl of milk will aggregate? This is driven by capillary forces where the meniscus of the Cheerio floating on the milk is curved upwards. The Cheerios clump together to remove this curvature that gives rise to an excess surface area.
We have learned how to control the meniscus from positive to negative by making the liquibots - which look like small open sacs and are only 2 mm in diameter - hang from the surface of the liquid and become heavier or lighter. So, the rim of the sac that is hanging goes up and down, depending on what we allow to diffuse into the sac.
Salt, for example, will make the sac heavier, and the meniscus changes from positive to negative. If we diffuse reactants into the sac, we can generate a gas that causes the sac to be pulled upwards. If the meniscus of the container that the sacs are hanging in is positive, as it is for water in a glass container, then, when the meniscus of the sac is positive, the sac is drawn to the walls. If we change the meniscus of the sac to negative, it is repelled from the walls.
By simply changing the sign of the meniscus of the sac, the sac can move to the walls, change the sign by loading things in the sac at the walls. It then moves away and dumps the loaded materials at a delivery site. The meniscus becomes positive and the sac goes back to the wall.
Very simply in concept, but oftentimes, the simple things are overlooked.
How does this new device compare to the family of robotic devices called ‘Liquibots’?
We have broken a barrier in designing a liquid robotic system that can operate autonomously by using chemistry to control an object’s buoyancy.
All current robotic systems require some external input. In the case of Liquibots, generally, magnetic particles are embedded in the robot, and 1D, 2D or 3D magnetic fields are required to move the robot. We demonstrated that the same could be done with our system, where we use an external magnet to move the sac across the interface.
However, in nature, even the magnetic field of the earth cannot move an object back and forth. The beauty of our system is that once the system is set, either diffusion of materials across an interface or a chemical reaction fed by reactants placed in the system from the start occur completely autonomously.
Granted, our motion is a simple back and forth motion, but doing this in more dimensions should be possible.
Did you come across any challenges during your research, and if so, how did you overcome them?
We did not really encounter any technical problems throughout our research. Once we understood the principles in play, it was merely a matter of honing the system to optimize a specific performance. It is, actually, a very simple experiment to realize.
According to your research, Liquibots can perform different tasks simultaneously depending on their formulation. What are some of these tasks?
The first task is continuous motion without any external input. The second is the transport of materials (chemical, charged polymer (electrolytes), or nanoparticle from one point in the system to another.
The sac can be laden with catalysts so that compartmentalized reactions can be realized. For that matter, catalysts can be picked up at one point and delivered to another point to stimulate a reaction at the drop-off site, so sequential reactions. One sees such behavior in the body where chemical reactions can be confined to one specific compartment and then feed reactions in another compartment.
We published a paper in Chem dealing with such behavior in all-liquid 3D printed systems.
Do you plan on working on solid surfaces rather than exclusively liquid surfaces?
The behavior we observed will only work with liquids.
What are the next steps for your research?
Next, we will focus on Aquabots - 3D printed all-aqueous systems where nanoparticles are embedded in the constructs. The all-liquid nature of these such 3D constructs should overcome the limitation in deformability currently plaguing soft robot systems made from silicon rubber-types materials.
We have a manuscript currently under consideration in Matter that follows on from some earlier work published in ACS Nano. We also discovered all-liquid magnets where we can produce all-liquid ferromagnetic materials (see X.Liu et al. below), and integrating this into the Liquibots or Aquabots would be really cool.
About Thomas P. Russell
Thomas P. Russell, the Silvio O. Conte Distinguished Professor of Polymer Science and Engineering at the University of Massachusetts in Amherst, received his Ph.D. in 1979 in Polymer Science and Engineering from the University of Massachusetts Amherst.
He was a Research Associate at the University of Mainz (1979-1981), a Research Staff Member at the IBM Almaden Research Center in San Jose, CA (1981-96).
He is also a Visiting Faculty at the Materials Science Division in the Lawrence Berkeley National Laboratory, an Adjunct Professor at the Beijing University of Chemical Technology, and a PI at the Advanced Institute of Materials Research at Tohoku University.
His research interests include the surface and interfacial properties of polymers, phase transitions in polymers, directed self-assembly processes, the use of polymers as scaffolds and templates for the generation of nanoscopic structures, the interfacial assembly of nanoparticles and wrinkling of thin polymer films.
He was the Director of the Materials Research Science and Engineering Center from (1996-2009), Director of the Energy Frontier Research Center on Polymer-Based Materials for Harvesting Solar Energy (2009-2014), a PI in the Global Research Laboratory at Seoul National University (2005-2015), and the Beijing Advanced Innovation Center on Soft Matter (2016-present).
He has over 1,000 publications, 35 patents and edited 5 books. He is a Fellow of the American Physical Society, Materials Research Society, Neutron Scattering Society of America, American Association for the Advancement of Science, and the American Chemical Society, Polymer Materials Science and Engineering Division.
He has received the Polymer Physic Prize of the APS, the Cooperative Research Award of the ACS, the Dutch Polymer Award, the ACS Award in Applied Polymer Science, Society of Polymer Science Japan International Award, the Shull Prize of the Neutron Scattering Society of America, and is an elected member of the National Academy of Engineering and the National Academy of Inventors.
Disclaimer: The views expressed here are those of the interviewee and do not necessarily represent the views of AZoM.com Limited (T/A) AZoNetwork, the owner and operator of this website. This disclaimer forms part of the Terms and Conditions of use of this website.