In the future, farmers could grow fruits and vegetables in multilevel warehouses with the help of more efficient methods for artificial pollination. This approach could significantly increase yields while reducing agriculture’s environmental impact.
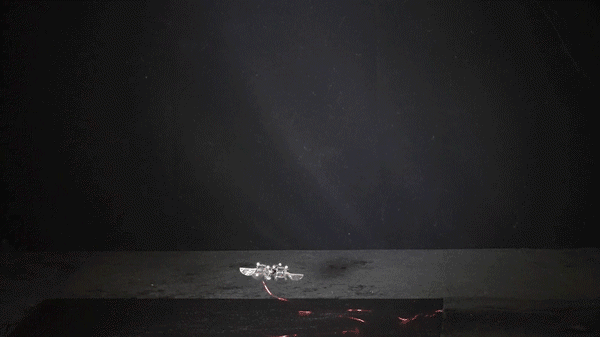
The robotic insect, weighing less than a paperclip, can fly significantly faster than similar bots while completing acrobatic maneuvers like aerial flips. Image Credit: Courtesy of the researchers
To turn this vision into reality, researchers at MIT are working on robotic insects that might one day emerge from mechanical hives to perform precise pollination tasks on a large scale. Despite advancements, even the most advanced insect-sized robots still fall short of natural pollinators like bees in terms of stamina, speed, and agility.
To address this challenge, the researchers have redesigned their robotic insects, drawing inspiration from the anatomy of real pollinators. The result is a new generation of tiny, flying robots that are significantly more agile and resilient than earlier models.
The latest robotic insects can hover for approximately 1000 seconds—over 100 times longer than previous models. Weighing less than a paperclip, these advanced bots also achieve significantly higher flight speeds and can perform complex aerial maneuvers, such as double flips.
The improved design enhances flight precision and agility while reducing mechanical strain on the wing flexures, resulting in faster movements, greater endurance, and an extended operational lifespan.
Additionally, the revamped design includes enough space to accommodate tiny batteries or sensors, paving the way for the robots to operate autonomously outside of laboratory settings.
The amount of flight we demonstrated in this paper is probably longer than the entire amount of flight our field has been able to accumulate with these robotic insects. With the improved lifespan and precision of this robot, we are getting closer to some very exciting applications, like assisted pollination.
Kevin Chen, Associate Professor and Study Senior Author, Massachusetts Institute of Technology
The paper was co-authored by Chen, alongside co-lead authors Suhan Kim and Yi-Hsuan Hsiao, both graduate students in Electrical Engineering and Computer Science (EECS). Additional contributors include EECS graduate student Zhijian Ren and summer visiting student Jiashu Huang. The research is published in Science Robotics.
Boosting Performance
Earlier iterations of the robotic insect consisted of four identical modules, each equipped with two wings, assembled into a rectangular structure roughly the size of a microcassette.
But there is no insect that has eight wings. In our old design, the performance of each individual unit was always better than the assembled robot.
Kevin Chen, Associate Professor and Study Senior Author, Massachusetts Institute of Technology
The reduced performance in earlier designs was partly due to the wing arrangement, where the flapping wings interfered with one another, decreasing the lift forces they produced.
The new design simplifies the robot by cutting it in half. Each of the four identical units now features a single flapping wing oriented away from the robot’s center, which stabilizes the wings and enhances their lift capabilities. By halving the number of wings, the updated design also creates additional space, allowing the robot to potentially carry onboard electronics.
The researchers also developed more advanced transmissions to link the wings with the actuators, or artificial muscles, responsible for their movement. These enhanced transmissions, paired with extended wing hinges, significantly reduce mechanical stress, addressing the endurance limitations of earlier models and making the robots more durable.
“Compared to the old robot, we can now generate control torque three times larger than before, which is why we can do very sophisticated and very accurate path-finding flights,” Chen said.
Despite these advancements, robotic insects still lag behind their natural counterparts. For example, a bee, equipped with just two wings, can execute rapid and highly controlled movements with remarkable precision, a capability that remains unmatched by even the most advanced robotic designs.
“The wings of bees are finely controlled by a very sophisticated set of muscles. That level of fine-tuning is something that truly intrigues us, but we have not yet been able to replicate,” he said.
Less Strain, More Force
The robotic insect's wing motion is powered by artificial muscles—tiny, soft actuators crafted from layers of elastomer sandwiched between ultra-thin carbon nanotube electrodes, then rolled into a flexible cylinder. These actuators compress and elongate rapidly, creating the mechanical force required to flap the wings.
In earlier designs, the actuators often buckled when operating at the high frequencies needed for flight, diminishing the robot's power and efficiency. The improved transmissions mitigate this buckling effect, reducing strain on the artificial muscles and allowing them to exert more force for wing flapping.
Another key enhancement is the addition of a long wing hinge that minimizes torsional stress during the flapping motion. Fabricating this hinge, which measures about 2 centimeters in length and only 200 microns in diameter, posed one of the team's biggest challenges.
If you have even a tiny alignment issue during the fabrication process, the wing hinge will be slanted instead of rectangular, which affects the wing kinematics.
Kevin Chen, Associate Professor and Study Senior Author, Massachusetts Institute of Technology
After numerous trials, the researchers successfully developed a multistep laser-cutting process to precisely fabricate each wing hinge.
With all four units integrated, the new robotic insect can hover for over 1,000 seconds—nearly 17 minutes—while maintaining consistent flight precision without any signs of performance degradation.
“When my student Nemo was performing that flight, he said it was the slowest 1,000 seconds he had spent in his entire life. The experiment was extremely nerve-racking,” Chen said.
The updated robot achieved an average flight speed of 35 centimeters per second, the fastest reported for this type of robotic insect. It also demonstrated advanced maneuverability, performing body rolls and double flips. Remarkably, it can even follow a programmed trajectory to spell out "M-I-T."
“At the end of the day, we have shown flight that is 100 times longer than anyone else in the field has been able to do, so this is an extremely exciting result,” he said.
Looking ahead, Chen and his team aim to further refine the design to enable the robots to sustain flight for over 10,000 seconds.
They are also working on enhancing the robots' precision, with the ultimate goal of enabling them to land and take off from the center of a flower. In the long term, the researchers hope to equip the robots with miniature batteries and sensors, allowing them to operate and navigate autonomously outside the lab.
“This new robot platform is a major result from our group and leads to many exciting directions. For example, incorporating sensors, batteries, and computing capabilities on this robot will be a central focus in the next three to five years,” Chen said.
This research is partially supported by funding from the US National Science Foundation and a MathWorks Fellowship.
Journal Reference:
Kim, S., et al. (2025) Acrobatics at the insect scale: A durable, precise, and agile micro–aerial robot. Science Robotics. doi.org/10.1126/scirobotics.adp4256.